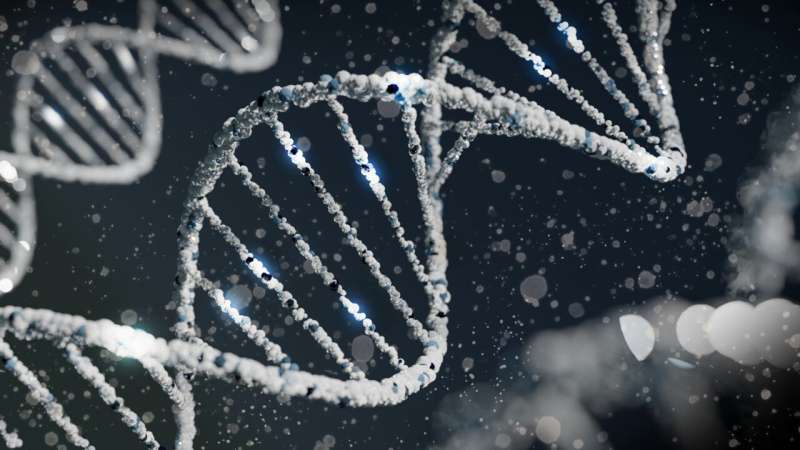
Genes make up the blueprints and outline the process of building every living organism. To ensure that the right genes are activated in the right cells, and in the right amounts at the right time, genes are constantly being regulated by small molecular machines made of proteins. When gene regulation fails, or specific genes are altered through mutation, the body is more predisposed to diseases such as cancer, Alzheimer’s, and autoimmune disorders.
Now, for the first time in animals, the lab of Daniel McKay, Ph.D., associate professor of biology in the UNC College of Arts and Sciences and of genetics at the UNC School of Medicine, has identified a crucial focal point in the regulatory processes that govern cell identity.
Researchers discovered that a chemical alteration on a single protein, histone H3, is essential for controlling the genes that help cells remember their own identity and function. When the genes and proteins in charge of keeping these regulatory processes in check are mutated, cells will proliferate out of control or resist cell death pathways, two key features of cancer.
Their results were published in Genes & Development. Cyril Anyetei-Anum, a Ph.D. candidate in the Curriculum in Genetics and Molecular Biology and member of the McKay lab, was lead author on the study.
The importance of gene expression and chemical modifications of histones
Our genomes are huge. Each cell contains over 6-billion DNA letters, that, if stretched out end-to-end, would measure 2-meters long. Small proteins called histones are tasked with tightly packaging all of that DNA into every cell, like a librarian shoving books into a full bookcase. Histones also play key roles in gene regulation.
Small chemical modifications can be added to histones, which allow proteins to access the tightly bound DNA from their histone bookcases to turn “on” and “off” genes in the organism. With ready access to DNA information, the body can carry out gene expression or convert genetic information into protein products that carry out actions specified in the DNA code.
Scientists have long studied the processes that underlie gene expression and the chemical modifications of histones because of the downstream effects they have on cellular processes.
One regulatory process of scientific interest is called epigenetic gene regulation. During this regulatory process, genes are altered in a “silent” manner, in that the genes are turned “on” or “off” without physically altering the DNA code. Scientists are intensely focused on epigenetic gene regulation because drugs can be developed to target specific histone modifications and switch specific genes “on” and “off.”
Methylation is one of the more prevalent chemical modifications that histones undergo. Researchers have previously found that the methylation of one particular location, lysine-4, on histone H3, is closely correlated with turning genes “on.”
Knowing that this relationship exists, researchers have built a hypothesis that histone H3 lysine-4 methylation is required for gene activation. However, it has been surprisingly difficult for researchers to test this hypothesis because the genetic tools did not yet exist to test H3 lysine-4 methylation in animals.
Evaluating histone H3 lysine-4 methylation in animals
Over the past 10 years, the McKay lab has been collaborating with several labs at the University of North Carolina at Chapel Hill to create an experimental animal model system they could use to study the role of histone proteins in regulating genes.
For this study, researchers examined Drosophila melanogaster, colloquially known as the fruit fly, because of its simple genetics and the fact that its genetic elements are incredibly similar to that of humans. With Drosophila as their animal model, McKay and colleagues first determined that the methylation of histone H3 lysine-4 is required for the activation of “master regulator” genes.
These genes are critical in the large-scale regulation of the early human body, including those that develop tissue and organ systems, produce specialized cells from stem cells, and aid cells in remembering their own identity and function.
If these genes are expressed at the wrong time or place, they can transform cells from one identity into another, which is one of the key features of diseases like cancer. Due to the distinctive power of these genes, their expression is tightly controlled, and layers of mechanisms exist to ensure they are turned “on” and kept “off” in the right times and places inside animals.
In addition to finding that H3 lysine-4 methylation is important for activation of master regulator genes, McKay and colleagues also found that it plays a key role in turning “off” the protein machines that silence master regulator genes. These gene silencing proteins, referred to as Polycomb complexes, play critical roles in helping cells remember their own identity.
Gene silencing and cancer
While every type of cell in our body expresses a specific set of genes that are required for its specialized role in the body, the cells are also using Polycomb complexes to “silence” genes that are not important for their predetermined role(s) at the same time.
When Polycomb complexes are turned “off,” impacted cells “forget” their identity and acquire new properties, like proliferating out of control or resisting cell death pathways, two key features of cancer cells. The findings suggest that drugs targeting histone H3 lysine-4 methylation may be effective in counteracting the effects of Polycomb mutations found in human diseases.
More information:
Cyril S. Anyetei-Anum et al, Evidence for dual roles of histone H3 lysine 4 in antagonizing Polycomb group function and promoting target gene expression, Genes & Development (2024). DOI: 10.1101/gad.352181.124
Citation:
Scientists discover new details of gene regulation that control cell identity (2024, November 26)
retrieved 26 November 2024
from https://medicalxpress.com/news/2024-11-scientists-gene-cell-identity.html
This document is subject to copyright. Apart from any fair dealing for the purpose of private study or research, no
part may be reproduced without the written permission. The content is provided for information purposes only.
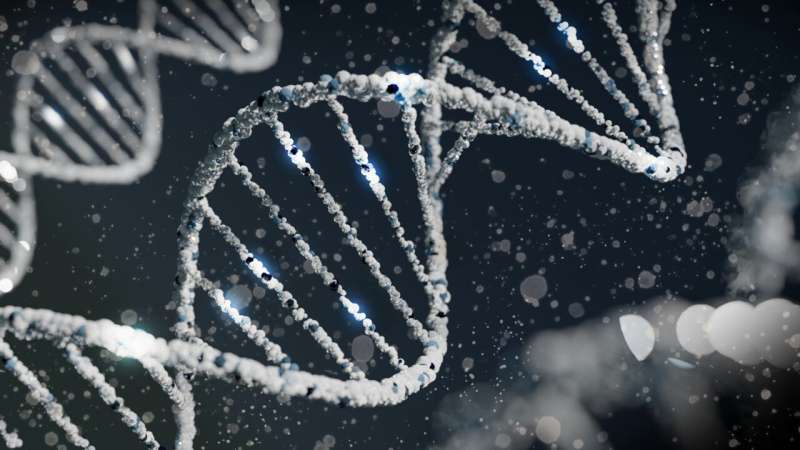
Genes make up the blueprints and outline the process of building every living organism. To ensure that the right genes are activated in the right cells, and in the right amounts at the right time, genes are constantly being regulated by small molecular machines made of proteins. When gene regulation fails, or specific genes are altered through mutation, the body is more predisposed to diseases such as cancer, Alzheimer’s, and autoimmune disorders.
Now, for the first time in animals, the lab of Daniel McKay, Ph.D., associate professor of biology in the UNC College of Arts and Sciences and of genetics at the UNC School of Medicine, has identified a crucial focal point in the regulatory processes that govern cell identity.
Researchers discovered that a chemical alteration on a single protein, histone H3, is essential for controlling the genes that help cells remember their own identity and function. When the genes and proteins in charge of keeping these regulatory processes in check are mutated, cells will proliferate out of control or resist cell death pathways, two key features of cancer.
Their results were published in Genes & Development. Cyril Anyetei-Anum, a Ph.D. candidate in the Curriculum in Genetics and Molecular Biology and member of the McKay lab, was lead author on the study.
The importance of gene expression and chemical modifications of histones
Our genomes are huge. Each cell contains over 6-billion DNA letters, that, if stretched out end-to-end, would measure 2-meters long. Small proteins called histones are tasked with tightly packaging all of that DNA into every cell, like a librarian shoving books into a full bookcase. Histones also play key roles in gene regulation.
Small chemical modifications can be added to histones, which allow proteins to access the tightly bound DNA from their histone bookcases to turn “on” and “off” genes in the organism. With ready access to DNA information, the body can carry out gene expression or convert genetic information into protein products that carry out actions specified in the DNA code.
Scientists have long studied the processes that underlie gene expression and the chemical modifications of histones because of the downstream effects they have on cellular processes.
One regulatory process of scientific interest is called epigenetic gene regulation. During this regulatory process, genes are altered in a “silent” manner, in that the genes are turned “on” or “off” without physically altering the DNA code. Scientists are intensely focused on epigenetic gene regulation because drugs can be developed to target specific histone modifications and switch specific genes “on” and “off.”
Methylation is one of the more prevalent chemical modifications that histones undergo. Researchers have previously found that the methylation of one particular location, lysine-4, on histone H3, is closely correlated with turning genes “on.”
Knowing that this relationship exists, researchers have built a hypothesis that histone H3 lysine-4 methylation is required for gene activation. However, it has been surprisingly difficult for researchers to test this hypothesis because the genetic tools did not yet exist to test H3 lysine-4 methylation in animals.
Evaluating histone H3 lysine-4 methylation in animals
Over the past 10 years, the McKay lab has been collaborating with several labs at the University of North Carolina at Chapel Hill to create an experimental animal model system they could use to study the role of histone proteins in regulating genes.
For this study, researchers examined Drosophila melanogaster, colloquially known as the fruit fly, because of its simple genetics and the fact that its genetic elements are incredibly similar to that of humans. With Drosophila as their animal model, McKay and colleagues first determined that the methylation of histone H3 lysine-4 is required for the activation of “master regulator” genes.
These genes are critical in the large-scale regulation of the early human body, including those that develop tissue and organ systems, produce specialized cells from stem cells, and aid cells in remembering their own identity and function.
If these genes are expressed at the wrong time or place, they can transform cells from one identity into another, which is one of the key features of diseases like cancer. Due to the distinctive power of these genes, their expression is tightly controlled, and layers of mechanisms exist to ensure they are turned “on” and kept “off” in the right times and places inside animals.
In addition to finding that H3 lysine-4 methylation is important for activation of master regulator genes, McKay and colleagues also found that it plays a key role in turning “off” the protein machines that silence master regulator genes. These gene silencing proteins, referred to as Polycomb complexes, play critical roles in helping cells remember their own identity.
Gene silencing and cancer
While every type of cell in our body expresses a specific set of genes that are required for its specialized role in the body, the cells are also using Polycomb complexes to “silence” genes that are not important for their predetermined role(s) at the same time.
When Polycomb complexes are turned “off,” impacted cells “forget” their identity and acquire new properties, like proliferating out of control or resisting cell death pathways, two key features of cancer cells. The findings suggest that drugs targeting histone H3 lysine-4 methylation may be effective in counteracting the effects of Polycomb mutations found in human diseases.
More information:
Cyril S. Anyetei-Anum et al, Evidence for dual roles of histone H3 lysine 4 in antagonizing Polycomb group function and promoting target gene expression, Genes & Development (2024). DOI: 10.1101/gad.352181.124
Citation:
Scientists discover new details of gene regulation that control cell identity (2024, November 26)
retrieved 26 November 2024
from https://medicalxpress.com/news/2024-11-scientists-gene-cell-identity.html
This document is subject to copyright. Apart from any fair dealing for the purpose of private study or research, no
part may be reproduced without the written permission. The content is provided for information purposes only.